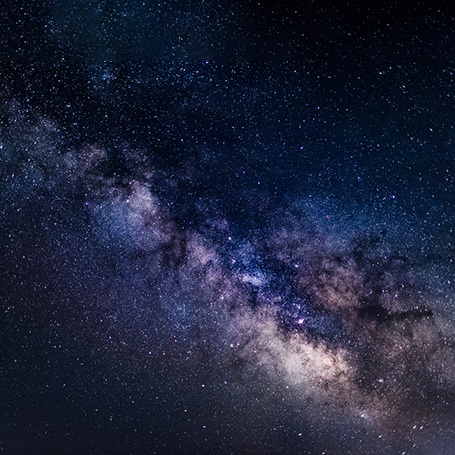
Peter Cabauy*, Larry C. Olsen, Tom E. Adams
City Labs, Inc., 301 Civic Court, Homestead, FL 33030
Purdue University, School of Nuclear Engineering, West Lafayette, IN 47905, USA
Naval Surface Warfare Center, Crane, IN 47522, USA
(*) – Corresponding author; peter.cabauy@citylabs.net, www.citylabs.net
Radiation interaction with materials can be used in a positive sense, such as converting kinetic energy into electricity. Though not a new concept, research has been minimal due to the limited low power applications, degradation, and cost. Betavoltaic cells are analogous to photovoltaic cells. The beta particle’s kinetic energy enters a p-n junction and creates electron-hole pairs. Subsequently, the built-in potential of the p-n junction accelerates the electrons and ions to their respective collectors. The major challenges are the beta source and electrical conversion efficiencies. The efficiency of the beta emitter is low due to self-shielding and isotropic emission. Electronic conversion is (at best) 10 percent, where voids and defects in the p-n junction reduce both the built-in potential and charge carrier mobility. Collisions of beta particles can also create unwanted defects and voids in the semiconductor material; silicon demonstrates this consideration especially well. Evaluating the effects of beta particle interaction with a p-n junction is the key to optimizing a betavoltaic cell design. A few techniques are proposed to improve the efficiencies and minimize material degradation.
Betavoltaic; Tritium; Hybrid; Hybrid Sources.
In 1953, Paul Rapport, working at RCA, was the first researcher to describe a betavoltaic device with a SR90-Y90 radioactive source. The device yielded an efficiency of 0.2%, but it degraded quite rapidly due to radiation damage [1]. Several others continued research into betavoltaics [2,3], but the most extensive effort in producing a viable betavoltaic power source appears to have been carried out at Donald W. Douglas Laboratories (DWDL) from 1968 to 1974; this successful endeavor was conducted under the leadership of Dr. Larry Olsen, a co-author of this article and a staff-member of City Labs, Inc [4,5]. In Olsen’s seminal betavoltaic work, Pm-147 beta sources were combined with custom designed Si n/p cells to produce nuclear batteries for cardiac pacemakers in over 100 patients during the 1970’s. Due to gamma radiation concerns, however, the Lithium battery was able to capture the pacemaker market.
Despite the stigma surrounding the earlier work, numerous research groups have continued their attempts to commercialize a safe, reliable betavoltaic battery. Subsequent thereto, Dr. Olsen published a betavoltaic review [6] suggesting Tritium as a safe, reliable, and abundant alternative to other radioisotopes. Independently, City Labs also concluded that Tritium would serve well as an excellent candidate for use within a betavoltaic battery design. This conclusion was prompted by Tritium’s existing use within the public domain where it finds applications in Exit signs for school buildings and theaters, glow-in-the-dark wrist-watch faces, and illuminated gun/rifle sights.
Researching betavoltaic devices requires a multidisciplinary background. A betavoltaic cell creates electricity similar to a photovoltaic or solar cell. In a betavoltaic cell, electrons are produced indirectly via the kinetic energy of the beta particles interacting with in the semiconductor. The basic concept of operation is shown in Figure 1. The beta particle enters the p-n junction and collides with atoms creating electron-hole pairs (EHP) as it slows down. A portion of the kinetic energy is lost to the lattice. A 5 keV particle could create 1000 or more EHPs, and those created near the intrinsic or depletion region contribute to the generated current collected at the contacts. The holes are accelerated to the p-side collector and the electrons are accelerated to the n-side collector. EHPs created outside the depletion layer quickly recombine and provide a net current of zero. With a load connected, the electrons travel from the n-side, through the load and back to the p-side. Because EHPs have relatively short lives, the voltage developed is a function of the semiconductor material and energy of the beta particle. The peak power is found by measuring the short-circuit current (Isc) and open-circuit voltage (Voc), which typically occurs about 0.75 V as shown in Figure 2.
Figure 1: Theory of operation of a betavoltaic cell.
Figure 2: Typical peak power of a betavoltaic cell.
Beta decay does not occur at specific discrete energies; rather the emitted electrons form a continuous energy spectrum which is different for each isotope. Figure 3 shows a generic beta energy spectrum. The average energy is typically one-third the maximum energy. Factors such as self-shielding and low enrichment reduce the quantity of betas that finally reach the betavoltaic converter device. The extent of self-shielding increases with isotope layer thickness and density. Another important factor is the penetration depth of the beta particle in the material. The range is a function of the material’s density and can be estimated using the following equation.
The overall conversion efficiency of a radioisotope power source is governed by three main characteristics: isotope emission efficiency, diffusion length, and semiconductor conversion. Beta emission is isotropic; therefore, over half of the beta particles never reach the semiconductor material.
Tritium betavoltaic power sources have been developed and demonstrated for long-life unattended applications in excess of 10 years. The design can provide continuous current (microamperes) over a wide temperature range (-60 °C to 125 °C) that no conventional chemical battery can match.
As shown in Figure 3, 220 day-old Tritium betavoltaic cells (NanoTritium™ batteries) were tested at Lockheed Martin and underwent thermal cycling from -50 ºC to 150 °C and exhibited no degradation. Future tests are planned for demonstration under MIL-STD-810 environmental conditioning. A regulatory general license is being obtained for the current NanoTritium™ battery designs, thereby providing the end-user with easy off-the-shelf utilization; this license designation subsequently negates any specialized end-user requirements for training and handling of radioactive materials.
Figure 3: City Labs’ NanoTritium batteries with relevant information on battery cover-plates.
City Labs’ NanoTritium™ battery demonstrates the highest combination of efficiency and energy density amongst the competing betavoltaic battery companies. This represents a considerable advancement in betavoltaic state-of-the-art and was confirmed by an independent third party industrial survey conducted by Lockheed-Martin [7]. City Labs’ Tritium betavoltaic cell operates through the principle of electron-hole pair generation within a proprietary semiconductor material; hole-pair generation is initiated by beta particle emissions supplied from a Tritiated metallic hydride foil. Each Tritiated foil/semiconductor couple provides approximately 0.1 μA/cm2 at 0.7 Volts. Encouraging data derived from prototype cells are presented in Table #1. Increases in voltage and current can be achieved via stacking of additional couples in series or in parallel.
Table 1: Prototype Data
Battery S/N |
Time (days) | VOC (V) | ISC (µA) | ISC (µA) @ T1/2 (12.3 y) Projected |
80005 | Initial | 0.7963 | 0.106 | 0.106 |
” “ | 43 | 0.7921 | 0.106 | 0.105 |
” “ | 58 | 0.7915 | 0.106 | 0.105 |
” “ | 77 | 0.7882 | 0.106 | 0.105 |
” “ | 86 | 0.7976 | 0.106 | 0.105 |
” “ | 220 | 0.7960* | 0.101* | 0.103 |
80006 | Initial | 0.784 | 0.118 | 0.118 |
” “ | 43 | 0.7785 | 0.117 | 0.117 |
” “ | 58 | 0.7781 | 0.117 | 0.117 |
” “ | 77 | 0.7793 | 0.116 | 0.117 |
” “ | 86 | 0.7865 | 0.116 | 0.116 |
” “ | 220 | 0.7737 | 0.113 | 0.114 |
80007 | Initial | 0.81 | 0.112 | 0.112 |
” “ | 43 | 0.8064 | 0.112 | 0.111 |
” “ | 58 | 0.8066 | 0.112 | 0.111 |
” “ | 77 | 0.8042 | 0.112 | 0.111 |
” “ | 86 | 0.8114 | 0.112 | 0.111 |
” “ |
220 |
0.7979* |
0.107* |
0.108 |
Combining this “continuous current charger” (i.e. betavoltaic) in parallel with a battery or capacitor can provide latent and burst power for many applications, e.g. unattended sensors, medical devices. A hybrid design will alleviate self-discharge losses at high temperatures.
In a hybrid design using both a NanoTritium™ battery and a lithium-ion battery, the Li cell would remain fully charged and last longer, even at high temperatures where the self-discharge can be 3% or more per month. In 6 days, a 1μA betavoltaic source could charge a 1.5 mAh Li-ion cell discharged by 10%. Therefore a 1.0 uA betavoltaic would provide an average of 7.0 mAh per year, which is sufficient to maintain a fully charged battery. The common lithium rechargeable coin cells (manganese, vanadium, niobium and titanium) have a narrower operating temperature range of -20ºC to 60°C. Niobium types do not require charging circuitry since they can be charged in a wide voltage range (1.8V-2.5V). Lithium coin cells are deemed safe and are exempted from the Navy’s lithium safety testing program. A design using super-capacitors (electric double-layer capacitors) will behave in similar fashion to the lithium design, but it will be more sensitive to temperature. The current technology exhibits leakage currents as low as 0.1 μA and an operating temperature range of –20ºC to 85°C. However, the life of the super-capacitors is limited by its operating temperature where the operating life doubles for every 10ºC decrease [8]. Super-capacitor voltages range from 2.3V to 5.5V. A stack of 4 to 8 betavoltaic cells (1-μA) would provide power and keep the super-capacitor fully charged at temperatures near or below ambient.
A hybrid combination of a NanoTritium™ betavoltaic cell trickle charging one of the above conventional secondary batteries can provide power burst capability for well over a decade, while providing consistent low current to other low power microelectronics. Anti-Tamper devices can protect against reverse engineering through hybrid betavoltaic trickle charging of a secondary lithium battery, such devices will provide long term, maintenance-free, high security to sensitive microelectronic military platforms. For remote sensing and RFIDs, the hybrid source would provide standby current and burst current capabilities to transmit and receive signals. The squibs of special application batteries, ordnance, and actuators could be remotely activated by a sensor powered by the hybrid design. Applications to commercialize the hybrid design lie in medical implants, oil-well logging, and real-time sensors.
Appropriate design of betavoltaic power cells must be accomplished through an understanding of and improvements to semiconductor device efficiencies. These cells demonstrate high potential for use in low power applications under a broad range of temperatures. The additional advantage afforded by these cells is the extreme long life-times that provide mitigated cost and logistics considerations; the immediate benefit, therefore, is the reduction of maintenance costs and tracking requirements for bringing systems offline and replacing power cells.
Ready to power your next innovation or learn more about our technology?
Contact Us Today